Frontiers
To inspire and educate—Salk’s Education Outreach is on a mission
Motivated by Jonas Salk’s admonition to “be good ancestors,” Education Outreach strives to inspire the next generation of scientists. By running programs like the Mobile Science Lab or High School Science Day among others, Education Outreach encourages scientific literacy, enthusiasm in science as a career, and awareness of the value of basic research.
Observations
Deepshika Ramanan—From iguana poop to breastmilk
Ramanan, a new assistant professor and microbiologist by training, made a chance discovery about animal gut bacteria that led to her fascination with breastmilk immunity.
Belonging
New strategic plan provides roadmap for enhancing diversity, equity, inclusion, and belonging at Salk
Groundbreaking scientific discoveries require talented people behind the scenes, and the more diverse those people are, the more groundbreaking their discoveries. At Salk, improving diversity, equity, and inclusion is a commitment to both scientific discovery and humanity.
Insights
Trinka Adamson—Supporting Salk science through animal care
Adamson, senior director of Salk’s Animal Resources Department, works to provide the highest quality veterinary medical care, husbandry, enrichment, and professional technical support to enable our scientists to conduct their animal research and make their bold discoveries.
President’s Letter
“Since the creation of the Institute, people have been the driving force of Salk’s scientific achievements. Jonas Salk sought out the brightest scientific minds—a cadre of Nobel Prize winners and other luminaries—to become the Institute’s first faculty.”
Discoveries
Spotlight
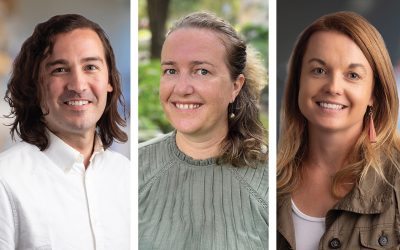
Salk adds three new faculty members
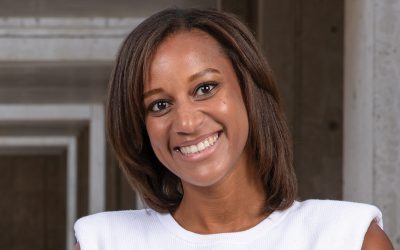
Assistant Professor Christina Towers receives NIH New Innovator Award
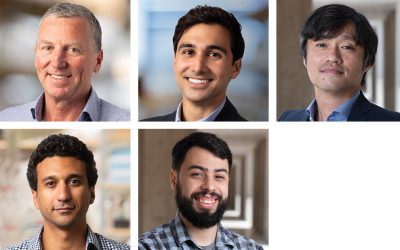
Investing in the future of innovation
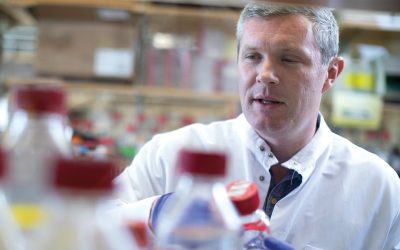
Physician-scientist Jesse Dixon named Rita Allen Foundation Award Scholar
Events
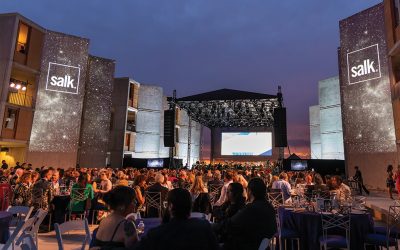
27th Annual Symphony at Salk
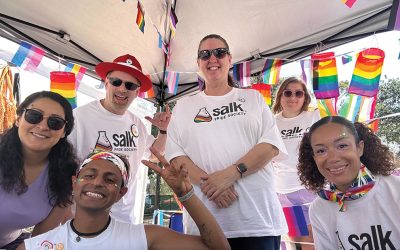
Full of Pride
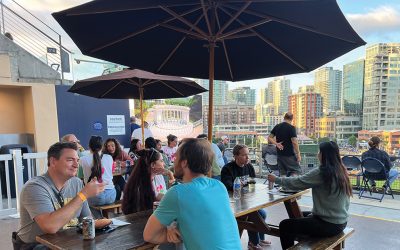
Alumni mixer and baseball night
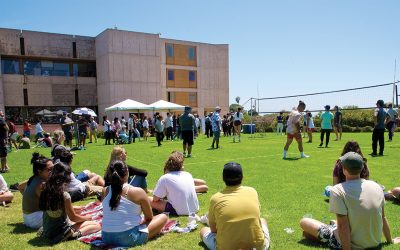